VM Tubes: Magnetrons and Similar Devices
VM Tubes: Magnetrons and Similar Devices
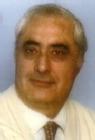
1 - Magnetrons
Tyne gives examples of early experiments on tubes where magnetic fields generated by solenoids control flow of electrons. De Forest and Von Lieben patented some kinds of such electron devices and Moorhead sold the A-P solenoid tube for a while.
Cyclotron type magnetrons
In 1921 A. W. Hull described for the first time the static characteristics of a device called magnetron (1). The tube had a linear filament in a coaxial cylindrical plate. The plate cylinder was surrounded by a winding, used to generate a magnetic field. The radial electric field and the superimposed magnetic field forced electrons to follow circular orbits before reaching anode. When the magnetic field was increased, radius of the orbits became smaller and smaller until electrons could not reach the plate, so causing a cut-off in the anode current. Hull also noted that adding a resonant circuit, just on the border between conduction and cut-off, the tube could sustain self-oscillations.
Fig. 1.1 – a) Draft of the Hull original diode. b) Diagram of the experimental circuit. c) CV2380 is a small-power X-band pulse magnetron intended to operate inside a cavity with a magnetic field of 2450 Oe.
The cylindrical anode magnetron oscillates in the so-called cyclotron mode, as the result of a sinusoidal voltage built up between cathode and anode. It was found that oscillation occurred following the relation λB = constant, where B was the intensity of the magnetic field. It was also noted that the period of oscillations was equal to electron transit time from cathode to anode and back. Since 1924 E. Habann proposed a split anode magnetron design (2), in order to have more stable oscillations at higher frequencies. In 1936, Cleeton and Williams reached frequency as high as 47 GHz with a split anode structure (3).
Negative resistance type
The split anode structure may also operate in a different mode, not depending upon the transit time, as far as it is small if compared with the period of oscillation. Under the effect of a very strong magnetic field, electrons follow spiral trajectories, executing several complete loops and being preferably attracted by the segment having the lower potential. In 1936 G. R. Kilgore succeeded in observing trajectories, made luminous by the introduction in the interelectrode space of some gas to permit ionization (4). Kilgore obtained efficiency as high as 25% at 600 MHz with 100W input power. In the '40s General Electric built split anode magnetrons, some water-cooled, capable of delivering about 150W at frequencies between 15 and 1200 MHz. Unfortunately no documentation can be found for this line of tubes. Some of them, 5J29, 5J30 and 5J32, are engraved as ‘Confidential’ on the glass.
Fig. 1.2 – a) Early split anode magnetron; b) Improved split anode structure; c) Complete oscillator, also showing the magnetic field generating coil, surrounding the glass bulb. d) 5J29 was a split anode magnetron manufactured by General Electric capable of generating 150W at 400 to 700 MHz; the tube was water-cooled through the hollow anode legs.
Microwave multi-cavity magnetrons (Travelling-wave type)
Fig. 1.3 – Early laboratory multi-cavity magnetron.
In 1934 Samuel at Bell Telephone had proposed multi-cavity structures (5), forerunners of high power magnetrons. The multi-cavity magnetron was subsequently investigated in Russia by Alekseroff and Malearoff. Between 1934 and 1935 K. Posthumus at Philips developed a four segment magnetron; Posthumus also left a theoretic treatment of the rotating electron clouds, which gave the relations between the tube geometry, as the number of anode segments, and the intensity of electrical and magnetic fields (6). In 1937 Herriger and Hülster recognized the phase-focusing by which electrons were synchronized (7). Nevertheless, until WWII magnetron was just physic laboratory oddness.
Around the late ‘930s radar applications in particular required high power pulses at considerably high frequencies. A multi-cavity magnetron structure was investigated in Great Britain to generate RF power at microwave frequencies. The first high-power microwave magnetron was assembled by J.T. Randall and H.A.H. Boot at Birmingham University and the prototype, sealed by wax, operated while continuously connected to the vacuum pump on 21st February 1940. RF bursts of about 500W were generated at about 3 GHz. GEC soon assembled early-industrialized prototypes of E1188 and then of E1189. The latter had been modified with a more efficient indirect-heated oxide-coated cathode. On 17th July a prototype of E1189 gave 12KW peak output pulses at 9.5 cm wavelength. Early prototypes all had six cavities, the anode blocks being machined using a revolver chamber as drilling template.
In August 1940 the design of E1189 was modified with 8 resonating cavities all around a larger cathode cylinder, giving the same out power with a smaller 6-lbs. permanent magnet.
Fig. 1.4 – From left: the prototype of the multi-cavity magnetron by Randall and Boot, the six-cavity anode block of early prototypes and one of the early E1189 production samples.
One of the E1189, the sample No. 12 and accidentally the first prototype with eight cavities, was brought by the Tizard mission to North America. Its design details were transferred to Western Electric, Raytheon and to MIT Radiation Lab. in U.S., and to R.E.L. in Canada. R.E.L. was the radar-manufacturing arm of the Canadian National Research Council. The development also continued in England, pushed by military. E1189 was later coded as NT98. E1198, later CV38, was a variant operating at 9.1 cm (8), (9), (10).
Early multi-cavity magnetrons showed erratic operations because of their tendency to jump from the fundamental pi-mode to other spurious modes. The strapping technique, devised in July 1941 at the Birmingham University, overcame the problem and pulse power was soon limited by the modulator power capability. The unstrapped E1198 gave about 12KW peak output power, with efficiency around 10%. The strapped variant was capable of generating about 120KW in output, with some 200KW in input. CV56 was the very early strapped magnetron to enter in production. Echelon strapping technique was soon followed by improved schemes, as double ring strapping, or by intrinsically stable multi-cavity anode structures, as the ‘rising sun’ below.
Fig. 1.5 – Examples of echelon and double-ring strapping and of rising-sun anode.
Very soon several types of multi-cavity pulsed magnetrons were available both in US and in Great Britain for frequencies up to 10 GHz and over, with output power ranging from some tens to several hundreds kilowatt or megawatts.
Fig. 1.6 – Bunching of electrons in 8-cavity magnetron. a) Draft shows the distribution of electric field inside the anode block in the π mode, so called because the phase difference between adjacent resonators is π. In the shown eight-cavity structure alternatively four segments are positive and four segments are negative. b) Draft shows the cloud of electrons inside the tube. When operating, the charges on the internal surface of anode segments follows the oscillations in the cavities behind, creating a clockwise rotating electric field. The magnetic flux lines are parallel to the cathode. Under the combined effect of electric and magnetic fields, electrons are bunched in four spokes directed time after time to the nearest positive anode segment, each electron following spiral trajectories. The operation resembles that of a synchronous motor.
===================================
- Pulse and CW magnetrons
Many multi-cavity magnetrons were designed for pulsed operation in radar applications. Multi-cavity magnetron is a complete self-contained source of RF energy, operating with no external resonating circuits. All it requires is a DC supply if CW, or a modulator to build the anode supply pulses. RF oscillations with continuous power in the order of kilowatts or peak powers of hundreds or millions watts are generated with fairly good efficiency. As general rule in pulsed magnetrons the duty cycle is usually around 0.1%.
Fig. 1.7 – Examples of pulse magnetrons. a) British CV56, derived from the unstrapped E1189, was the first strapped magnetron to enter in production in the late 1942. Peak power increases from 12KW to about 100KW while more than 40% efficiency was obtained. b) This is one of the many US types directly derived from the British design, with the addition of two lateral mounting brackets. The specimen in the picture is a 706EY, capable of delivering 200KW output pulses. c) The popular 12-cavity, double ring strapped X-band magnetron 725A was designed by Western Electric scaling down the design of S-band types. It originated many variants, as 730A, 2J49 or 2J50. d) 725A also originated British variants, as this CV208, designed by GEC as plug-in replacement for CV108. e) 4J52A was an integral magnet X-band magnetron capable of generating pulses in the order of 80KW. f) CV513, British equivalent of 4J53, generated 900 KW pulses at 2803 MHz. g) 7503 is a baby X-band magnetron for beacon applications, 200W peak.
- CW magnetrons
CW magnetrons are available in a variety of types intended for ECM, RF heating and diathermy: with the exception of special types for ECM, they operate at fixed assigned frequencies with output power ranging from hundreds watts to some kilowatts. Common microwave ovens use a CW magnetron as source of heating radiation. Differences against pulsed magnetrons are in the anode geometry, modified to operate at lower anode voltage, and in the cathode emission characteristics.
Fig. 1.8 – Some CW magnetrons. Even if the tunable QK-60 (a) is given for pulsed operation, pulse length can be considerably extended or repetition rate can be increased. It is similar to the fixed frequency 5609 (b), 70W output at 2450 MHz, intended for RF heating. Both probably derived from the experimental CW type CM16B described by Collins. German MG8/200 (c) is capable of delivering 250W at 2.42 GHz.
--------
Another magnetron family includes the so-called VTMs, voltage tuned magnetrons. Here the anode has an interdigital vane structure: bunching effect does not depend upon the Q of resonating cavities and it is difficult to obtain in the presence of a normal space charge. The problem has been solved by the use of a virtual cathode, a deflector that launches in the interanode space a limited number of electrons injected from a traditional cathode placed elsewhere in the tube.
Fig. 1.9 – ZM-6086, CW voltage tunable magnetron, and draft of the virtual cathode used to facilitate electron bunching.
Other odd magnetrons were developed for special applications, beacons, guidance, ECM equipment or even UHF television tuners. Some of them require external resonating lines or cavities. Also a dual port magnetron was devised, called Amplitron or Platinotron, operating essentially as a backward-wave amplifier with electronic regenerative feedback.
-------------
Reference
1) A. W. Hull, ‘The Effect of a Uniform Magnetic Field on the Motion of Electrons Between Coaxial Cylinders’, Phys. Rev. Vol. 18, 1921.
2) E. Haban, ‘Eine neue Generatoröhre’, Zeits. F. Hochfr., Vol. 24, 1924.
3) C. E. Cleeton and N. H. Williams, ‘The Shortest Continuous Radio Waves’, Phys. Rev., Vol. 50, 1936.
4) G. R. Kilgore, ‘Magnetron Oscillators for the Generation of Frequencies Between 300 and 600 Megacycles’, Proc. I.R.E., Vol. 24, August 1936.
5) A. L. Samuel, U.S. Patent 2,063,342; December 8, 1934.
6) K. Posthumus, ‘Oscillators in a Split Anode Magnetron’, Wireless Engineer, Vol. 12, 1935.
7) F. Herriger und F. Hülster, ‘Die Schwingungen der Magnetfeldröhren’, Hochfreq. Und Electroak., Vol. 49, 1937.
8) George B. Collins, ‘Microwave Magnetrons’, M.I.T. Radiation Laboratory Series, BTP Edition, Lexington, Mass.
9) E. B. Callick, ‘Metres to Microwaves’, P. Peregrinus Ltd.
10) E. G. Bowen, ‘Radar Days’, Adam Hilger, Bristol
========================================================
2 - The Trochotron
The trochotron was an exotic counter based upon the interactions of electrons with combined electric and magnetic fields in a special electrode structure. For some aspects it recalls the principle of a multi-cavity magnetron. To understand its operating principle we refer to the figure below, illustrating the internal structure and the connections of a typical count-by-10 tube.
Fig. 2.1 – Electrode structure of a divide-by-ten trochotron. Parts of the supply busses are also shown.
An inner ring of 10 V-shaped spade electrodes, S0 to S9, surrounds the central cathode; other 10 electrodes, called targets, are all around, each target partially shadowed by the corresponding spade. Between each target and the successive spade there is a grid rod; all odd grids are connected together to a base pin and all the even grids are connected to another pin. Target supply voltage is in the order of +250V, while spades are connected to a considerably lower voltage supply, typically around +55V. The envelope containing the described electrode structure is immersed inside a cylindrical magnet, flux lines being parallel to the cathode itself.
This tube has several operating modes, some oscillating too. The theory was quite complex and poorly documented, but here we just want to give an intuitive description of its operation as counter. At the power-on electrons coming out from the cathode are attracted by the spades all around the inner ring. Under the combined influence of the electric field generated by the spades and of the external magnetic field, electrons follow spiral trajectories before being uniformly attracted by the 10 spades. Due to their curved trajectories and to the shape of spades, any influence by the targets behind is masked off.
The initial counting conditions usually can be set by temporarily lowering the potential of a given spade. Spade S0 voltage must be lowered for a short while, to perform the initial zero set. Electrons before directed to spade S0 can now feel the influence of the electric field generated by the target T0 behind. The flow, before directed to S0, now reaches almost entirely the target and only a small current, less than 15%, still flows through the spades before. The current distribution to the target T0 and the spade S0, after the zero reset, is roughly illustrated by the dashed path starting from the cathode in the figure above. This is a stable state and the current through the selected target can be considered near to constant, in the order of one to few milliamperes.
The switching grids are driven by a bi-phase sequencer, which generates negative pulses alternately on the even and on the odd grid groups. A negative pulse of suitable amplitude applied to the grid close to the holding spade counteracts the attraction of the selected target and releases the beam from the target itself. Repelled electrons, due to the rotating trajectories imposed by the magnetic field move to the leading spade. The current increase through the leading spade, cause a potential drop and the beam is then attracted by the corresponding leading target electrode. A new stable condition is then reached for each counting pulse.
Of course the magnetic field influence the trajectories of electrons. Its intensity depends upon the geometry of electrode structure and is related to the working voltages. The supply voltages must be stable within the prescribed values and good flux stability is also required. To grant predictable operation, usually the magnet was factory supplied, already installed.
The trochotron evolved from the many studies carried out at the time on beam switching devices. Its principle had been devised at the Research Division of Burroughs Adding Machine Co. as coding tube for high speed PCM applications. A four-page article was given in the November 1953 issue of Electronics. Here a binary coded counter was used to generate the magnetic deflection of a ribbon beam data selector.
Haydu Brothers, a small tube manufacturer of Plainfield, New Jersey, in progress of being fully owned by Burroughs itself, was someway involved since the manufacturing of prototypes. Haydu Brothers advertised the first commercial trochotron in the May 1955 issue of Electronics. It was claimed to replace 152 components, including 22 vacuum tubes. The tube, first named MBS, acronym for Magnetron Beam Switching tube, was later registered as 6700.
In December Haydu also advertised a complete counting module, the ‘Vari-Count’. It used the 6700 trochotron and another Haydu component: the HB106 Nixie 10-digit indicator, directly driven by the counter. Here are three ads published since May 1955 in Electronics.
Later Haydu entirely merged with Burroughs and tubes came with the latter mark. Here you find more information about roles of Burroughs and Haydu in developing and manufacturing the trochotron and the Nixie indicator.
As said before, the first commercial trochotron was the 6700, an unshielded, external magnet counter. The tube could not be mounted close to magnetic materials or other counters. 6703, also known with its proprietary code BD-301, was a shielded version. It was bulky, since the magnetic shield had to be mounted well spaced from the permanent magnet.
In order to reduce the size of its counters, Burroughs introduced a new family of trochotrons, the Beam-X family, with the magnet source moved inside the electrode structure. Here 10 small magnetic rods were placed behind the 10 spades, where they could also act as target electrodes, as in the picture below. Both unshielded and shielded versions were introduced; see BX1000 and BX2000 series.
Fig. 2.2 – Internal structure of a Beam-X counter.
Fig. 2.3 – From left: 6700 was the first trochotron commercially available. 6703 was the shielded version; in this picture from the bottom are visible both the magnet and the well-spaced outer shield that surround the glass bulb. Burroughs moved magnets inside the electrode structure in the Beam-X family: in the BX1000 or 6710 on the right, magnetic rods can be seen through the glass body. The family also includes shielded types.
Trochotrons found several niche applications in high-speed top priced counting equipment. These devices were bulky and expensive when compared with other counters, as the Ericsson dekatron, but capable of operation at frequencies as high as 10 MHz, 500 times faster than say a 'fast' GC10/D.
========================================================
3 - The Phasitron
The phasitron is a vacuum tube designed to operate as phase modulator in FM transmitters, that uses the interactions of electrons with combined electric and magnetic variable fields. It is not a VM tube, nevertheless it deserves few words because of the ingenuity of its design. Robert Adler of Zenith Radio first introduced the device in January 1946. In the years General Electric produced a couple of tubes based on its operating principle and used in commercial FM transmitters.
The structure of this tube generates a rotating undulate electron disk, as in the figure below.
Fig. 3.1 – Perspective view of the ruffled electron disk, deflected by the radial deflecting rods.
Electrons emitted from the cathode are first focused in a sharp-edged disk and then pass through a deflection system, formed by two parallel circles of radial rods, connected into three interlaced groups and driven by a 3-phase RF source. As result, electrons are forced to move as a rotating undulate disk that is intercepted by the anode system formed by two coaxial anodes, anode No. 1 having twelve holes punched above the plane of the disk and twelve holes punched below. Due to the holes in the first anode, from time to time the rotating electron disk impinges on the first anode or, passing through the holes, reaches the second anode. The current flowing to the second anode will be sinusoidal at a frequency related to the one driving the deflecting system. Now, if a variable magnetic field is applied perpendicularly to the plane of the electron disk, an angular displacement is introduced in its rotation, causing a phase shift in the output current.
In the actual phasitron, just one set of radial rod is used, the upper one being replaced by a neutral plane. The modulation coil is mounted all around the glass envelope of the tube. A cut-away view of the tube, mounted inside the coil and connected to the external circuits, is shown in the figure below.
Fig. 3.2 – The phasitron electrode structure with connections to external circuit blocks.
Fig 3.3 - Pictures of the General Electric GL-5593 Phasitron.
To thank the Author because you find the post helpful or well done.
The Platinotron or Amplitron
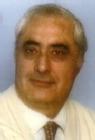
Platinotron, also called Amplitron, is a microwave high-power amplifier introduced by Raytheon around 1957. It was originally proposed to build add-on equipment for existing radar sets, to increase their search range: more or less as a linear amplifier does when added to a 200W transceiver. The platinotron looks like a multicavity magnetron and like a magnetron it requires a transverse magnetic field. But in its operation it also recalls a TWT because of the interactions between electron spokes and a wave moving along the internal transmission line.
Fig. 1.2.1 – Two views of the QK520 platinotron. The first one shows the assembled unit, complete with the magnet. Coolant flows through the two pipe fittings between input and output connectors. The second picture shows the QK520 with a cover removed. Small copper ducts connect anode vanes to the outer cooling rings.
Fig. 1.2.2 – Internal schematic of the QK520 platinotron. It is very similar to any vane anode magnetron. The only difference is the bifilar ring line from the input to the output port: odd vanes are all connected to one ring, even vanes to the other. A rotating four-spoke cloud of electrons is also represented around the cathode. Not visible in this figure the external magnetic field with flux lines parallel to cathode.
When RF is applied to the input port, it propagates through the bifilar line to the connected vanes that form the anode structure, up to the output. Now a voltage pulse with a controlled ramp-up is applied between cathode and anode. Under the combined effect of electric and magnetic fields electrons start to rotate. Their angular velocity increases with the ramp-up of the voltage pulse, until peripheric electrons rotate synchronously with the propagating signal wave. At this point electrons are bunched in rotating spokes, as in any other cavity magnetron. Rotation speed is locked on the propagation speed of the input signal regardless of any further voltage increase between cathode and anode, that only causes a subsequent increase in the current flow. Energy is then transferred from electrons to the signal.
As amplifier the platinotron shows peculiar behaviors. It is a saturated amplifier rather than a linear type. As evidenced by the following figure 1.2.3(a), it does not operate until the input signal rises over a given threshold: clearly if the electric field between anode vanes is too low no bunching occurs. Once locked on the input signal, the magnitude of the RF output depends almost exclusively on applied DC power. Input signal has just some influence on the efficiency, that increases at high drive levels. Input power is almost entirely transferred at the output port, added to the output of the platinotron amplifier. Other important features are the wide bandwidth and the efficiency of this amplifier. Platinotron locks on input signals over a typical 10% bandwidth, with efficiency from 50 to 70%.
Fig. 1.2.3 – (a) Plot of the output versus input power at different supply levels. (b) Typical performance chart at different magnetic field levels.
In typical operation as radar amplifier QK520 can be pulsed to 40 KV at 35 A. Driven by 40 KW input pulses it gives 800 KW in output. Efficiency is over 55% from 1225 to 1350 MHz.
Of course platinotron can also be operated as oscillator, as in the backward wave oscillator, with a reference cavity to stabilize frequency.
To thank the Author because you find the post helpful or well done.